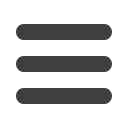
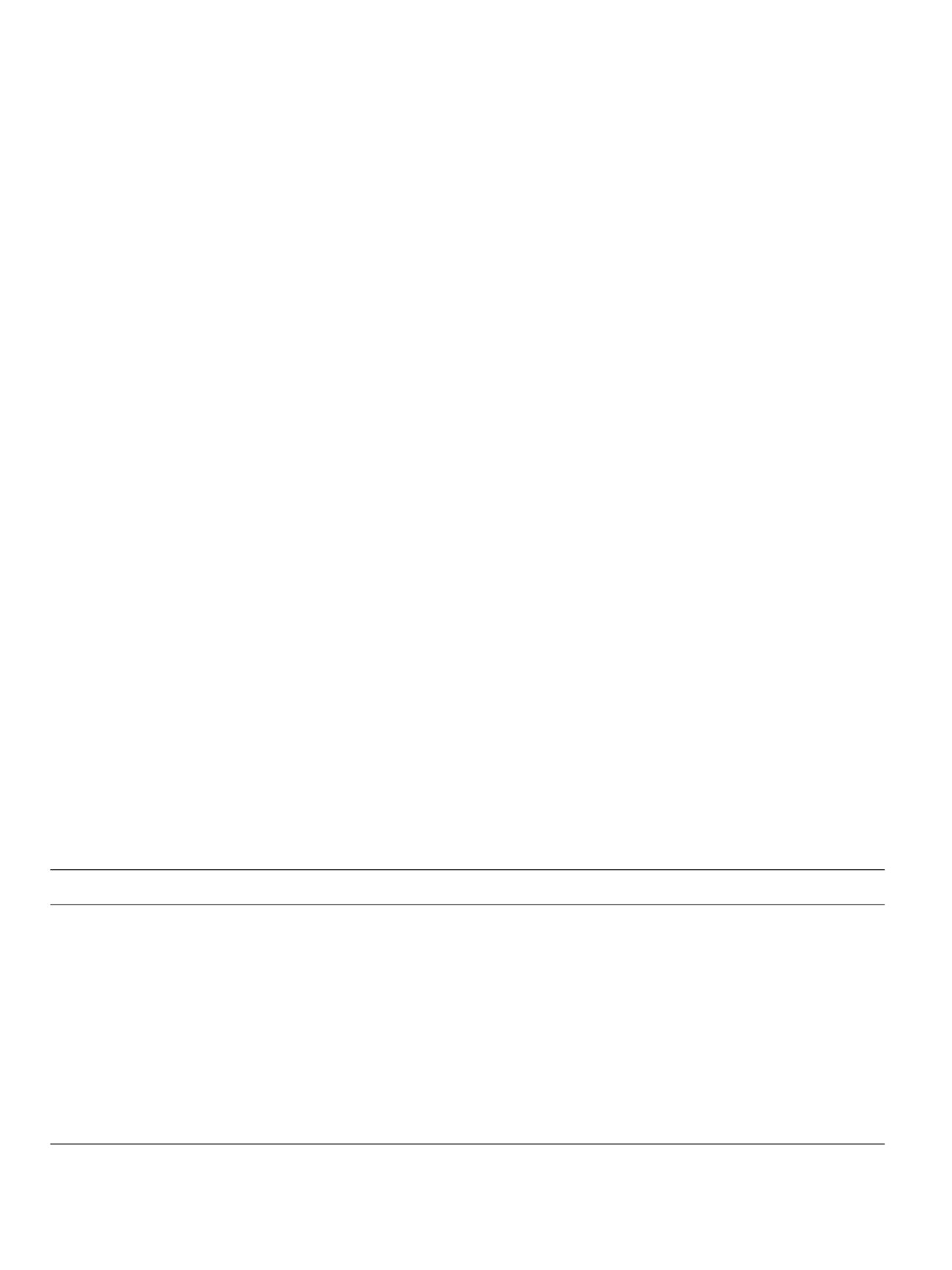
In order to determine the risk of a fracture to occur the concept of factor
of risk was introduced. The factor of risk can be computed as the ratio
of applied load and load at which the bone structure would fail [6].
In osteoporosis bone mass is reduced and the microarchitecture of
bone is deteriorated leading to enhanced bone fragility and increased
fracture risk [7]. The reduction in bone mass mainly results from
increased bone resorption and inadequate bone formation leading to
a negative remodeling balance [8]. Although less well understood,
also the intrinsic material properties of bone tissue are affected
by aging and osteoporosis [9]. Intrinsic changes that have been
previously described include compositional factors such as mineral-
ization distribution, content of collagen and cross linking profiles of
inter- and intrafibrillar collagen connections [10].
Aging and osteoporosis affect elastic properties as well as strength
properties of bone. Elastic properties describe the deformation which
occurs under loading (stiffness) before failure, while strength describes
the stress (force per unit area) at which failure occurs. For cortical bone,
stiffness decreases by 1
–
2% per decade and strength decreases by 2
–
5%
per decade [11]. Most importantly the energy required to fracture a
bone may decrease by up to 10% per decade beyond the age of 35 years
[6,12]. For trabecular bone the mechanical competence is mainly
determined by the apparent density and the orientation of the
trabecular network, explaining up to 90% of its variance [13,14]. As
the relationship of density with mechanical properties is non-linear,
the decreasing apparent density of trabecular bone with aging is
associated with accentuated deterioration of the mechanical pro-
perties. At age of 80 years the strength of the bone from the proximal
femur is reduced by more than 50% from its strength at young age [15].
Even more pronounced is the loss of mechanical strength at the spine
were the strength reduction during lifetime has been reported to
amount to up to 70% [16]. As the load to fracture for a whole bone
depends on both cortical and trabecular bone material properties the
overall strength of bone is dramatically reduced with aging. The
proximal femur loses about 50% of its strength and 70% of its energy to
failure between the age of 35 years and 75 years [17]. Even more
dramatic is the loss of strength at the spine where a loss of 80% of
compressive strength have been reported in men and women [18].
These dramatic age related changes in the material properties indicate
that the factor of risk for fracture is increased and traumatic events
which are benign at young age will become enormously hazardous in
the elderly.
Considering the concept of factor of risk for a fracture not only the
strength of the bone but also the applied load has to be taken into
account. With aging muscle performance and coordination deteriorate
and lead to an increased risk of falling and also to a decreased ability to
support falls. The potential energy which is generated during a fall
from standing height largely exceeds the energy required to fracture
the proximal femur. Thus without any energy absorption by soft tissue
dampening, muscle contraction or compensatory movement, the load
acting on the proximal femur during falling would inevitably lead to
hip fracture [19].
Failure of fracture fixation
Failure of internal fixation in osteoporotic bone typically results
from bone failure rather than implant breakage [20]. The deterioration
of cortical and trabecular bone with aging and osteoporosis goes along
with a considerable reduction of fixation strength of osteosynthesis
materials [21]. This reduction in fixation strength has been demon-
strated for most types of osteosynthesis materials including screws,
plates, nails and fixators (Table 1). It appears that at locations which are
prone to osteoporotic fractures also the effect of bone density on
fixation stability is most pronounced. In cortical bone; in which the
extent of deterioration of bone mechanical properties with age is less
pronounced, the thickness of the cortical bone has shown to have a
dramatic effect on the fixation stability of osteosynthesis implants
[22,23]. Compared to thick cortices the holding force decreases by
1000 N (or 50%) per 1 mm loss of cortical thickness. This might
generate differences in holding power of bone screws of up to 2000 N
within an individual bone and highlights the importance of placing
bone screws in the bone with thick cortices wherever possible.
The role of locked plating
It is generally assumed that locking plate constructs have
mechanical advantages compared to conventional plate constructs
and that these advantages are of particular benefit in osteoporotic bone
[20,34]. Biomechanical studies so far have demonstrated that in
osteoporotic bone locking plates create increased fatigue strength
and increased ultimate failure loads compared to conventional plates
[35,36]. Furthermore; it appears that the fixation stability of locked
plates is less susceptible to reduction in bone mineral density
compared to conventional plating constructs (Table 1). The major
reason for failure in conventional plating of osteoporotic bone is break
out of the screws and/or fracture of the bone through one of the screw
holes. Thus the stress within the bone at the site of the screws appears
Table 1.
Loss of mechanical properties for osteosynthesis constructs related to age and osteoporosis
Type of implant
Location
Loading mode
Mechanical property
Loss in mechanical
property (%)*
References
Pedicel screw
Vertebrae cervical
Axial screw pull out
Failure force
37
[24]
Screw tightening
Failure torque
35
Vertebral body
replacement
Vertebrae lumbar
Axial compression
Force
55
–
75
[25]
Cage & Fixator
Vertebrae lumbar
Flexion/Extension
Stiffness (1/ROM**)
60
–
80
[26]
Pedicle Screw
Sacrum (S1)
Cantilever bending
Failure force
64
[27]
Conventional plate
Tibia proximal
Tibial plateau
compression
Failure force
40
[28]
Conventional plate
Tibia distal
External rotation
Failure torque
70
[29]
Locking plate
14
Locking screws
Tibia shaft
Axial pull out
Failure force
15
[22]
Cantilever bending
Failure force
18
Cancellous screws
Humerus head
Axial pull out
Failure force
18
[30]
Conventional plate
Humerus proximal
Cyclic fatigue
Cycles to failure
70
[31]
Locking plate
59
Hip screw
Femural head
Cyclic fatigue
Stiffness (1/subsidence)
55
[32]
Proximal femoral nail
Femur proximal
Cyclic fatigue
Cycles to failure
48
[33]
*Loss in mechanical property was calculated as percentage reduction observed for the low density (osteoporosis) group or population with respect to the high density (normal bone) group or
population.
**ROM: Range of motion.
C. von Rüden, P. Augat / Injury, Int. J. Care Injured 47S2 (2016) S3
–
S10
S4