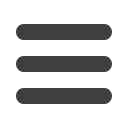
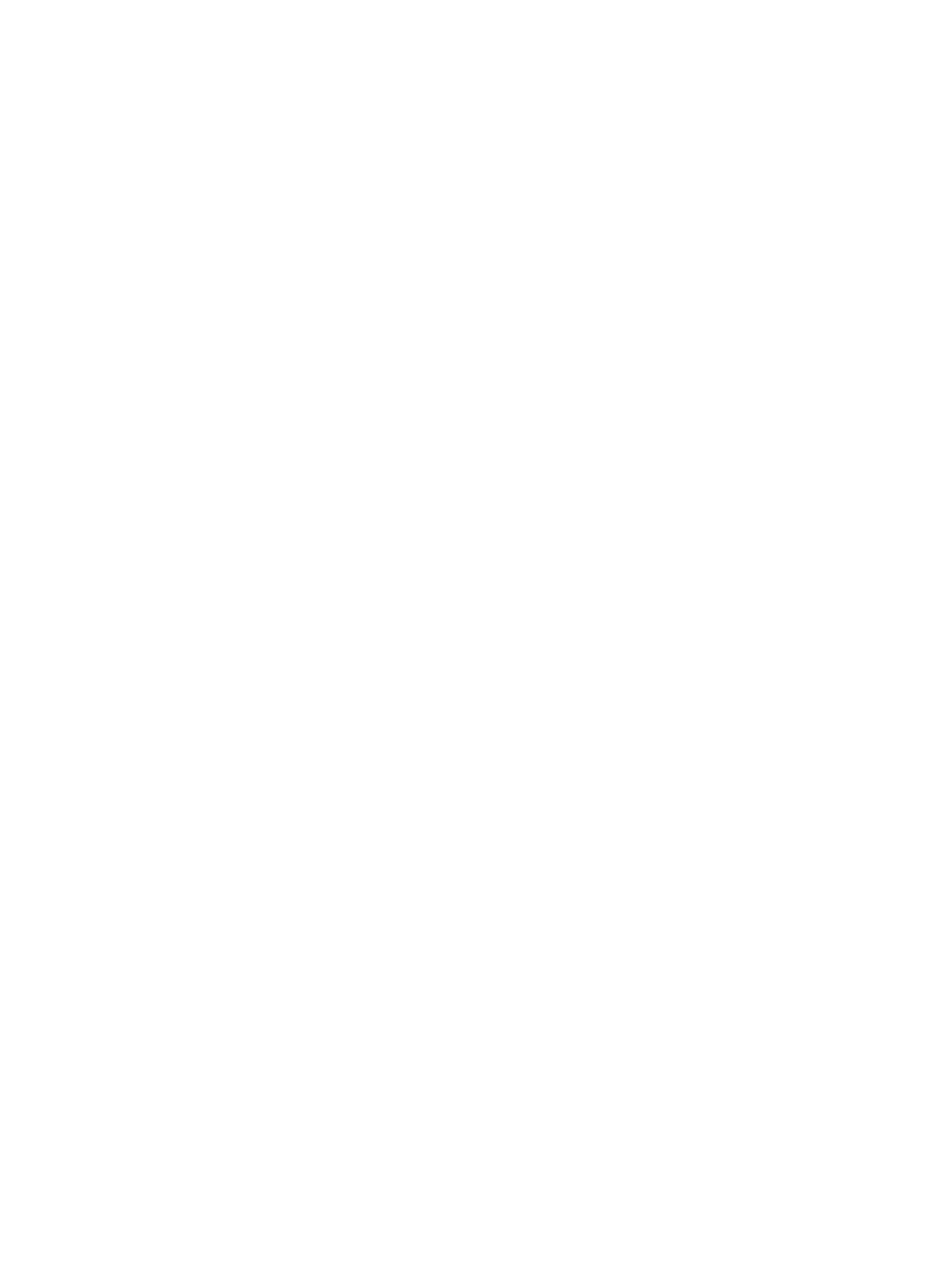
to be of importance for fixation failure. The major difference between
locking and conventional constructs is the load transfer between
fracture fragments. Conventional plates rely on frictional load transfer
between the plate and the bone. Thus loads are transferred from the
bone to the plate across the fracture area and back to the bone again. If
the applied load to the fractured bone exceeds the frictional force, the
construct becomes unstable mainly because the bone screws begin to
toggle due to shear [34]. Friction in conventional plating is produced by
compressing the plate on to the bone by tightening the compression
screws. This compression induces a considerable amount of preload in
the bone tissue around the screws which further increases the risk of
screw break out.
In locked plating the plate is not compressed on to the bone surface
and load transfer from the bone to the plate is always achieved through
the head of the locking screw. The load transfer from the bone to the
screw is distributed along the length of the screwwherever the screw is
in contact with bone. Furthermore, the locking mechanism of the
screw within the plate prevents individual screws from toggling in the
bone and cutting through the bone by cyclic fatigue. As there is no
compressional force during plate application in locked plating; the
bone around the screws experiences very little preload in the absence
of physiological loading.
In a recent computational study the stresses within the bone
around the screws have been computed for conventional plates and for
locking plates [37,38]. It has been shown that in osteoporotic bone
locking plates indeed demonstrate clinical benefit by producing
considerably lower tensile strains in the bone around the bone
screws. This provides a mechanical explanation for the improved
performance of locking plates in poorer bone quality and explains
previously reported higher incidence of screw loosening using the
conventional plates [39]. In good quality bone however, locking screws
caused similar strains to conventional screws and did not show much
mechanical advantages, suggesting that simple fractures in healthy
bone should be treated with reduction and absolute stability using
conventional plate constructs [37]. Finally, compared to conventional
plates locking screw constructs are less likely to fail by screw breakage
or screw loosening. Locking screws typically possess a thicker core
diameter and thus provide increased bending stiffness and strength. If
correctly locked into the plate screws rather break off at the screw plate
interface but do not become loose from the plate [40].
Treatment of fragility fractures
The treatment of osteoporotic fractures is determined by three
main factors: The soft tissues, the fracture configuration, and the
patients
’
status. In elderly patients, each of these three factors
may present particular problems [41] as thin soft tissues and skin
due to atrophy or malnutrition, ischaemic changes and poor healing,
oedema, ulcers and chronic skin lesions. Fracture configuration is often
comminuted, and even patient factors are often complex in the elderly,
because the majority of patients have also medical comorbidities
which require careful treatment.
The aim of surgical acute care after fragility fracture in the elderly is
a fracture management with stable fracture fixation facilitating early
full weight bearing. Compared with younger patients, elderly patients
do not tolerate pain, blood loss, immobilization, surgical mistakes, and
operative revisions. Mental condition and functional requirements of
the elderly patient strongly influence the decision for operative
treatment of the fragility fracture. It is important to notice, that the
overall complication rate and mechanical implant failure following
surgical treatment of fragility fractures are significantly higher
compared with non-fragility fractures [42]. As demonstrated earlier
in this manuscript osteoporotic/aged bone is the main cause of failure
of fracture fixation rather than implant failure itself. Complication rates
after surgical therapy of osteoporosis-related fractures are twice as
high as after treatment of healthy bone. The implant related failure rate
in osteoporosis-related fractures is estimated to be about 10
–
25% [1].
Surgical treatment of these fragility fractures is associated with a
higher rate of complications as mal- or nonunion [43]. Surgical success
is based on the correct indication as well as on the correct surgical
technique (
“
surgeon factor
”
), biological factors (e.g. perfusion of
fracture fragments) and on biomechanical factors (e.g. bone quality,
fracture configuration, anatomical reduction). Also patients
’
collabor-
ation during the postoperative care (
“
patient factor
”
) is a mandatory
prerequisite for sustainable success of the therapy.
It is common consent from epidemiological studies that persistent,
non-treated osteoporosis significantly increases the risk for another
fracture [44] and aggravates fracture fixation in various implants
e.g. single screws, screw-plate constructs, intramedullary nails or
dynamic hip screws at different bone locations as proximal humerus,
proximal femur or vertebra under different loading modes as quasi-
static or limited cyclic [21].
Principles of fracture fixation in osteoporotic bone
Techniques of open reduction and internal fixation (ORIF) have
commonly been developed for normal healthy bone. In osteoporotic
bone it is paramount to consequently apply these techniques.
Sometimes it might be necessary to modify traditional techniques in
order to avoid fixation failure and achieve satisfactory healing results
[1]. Fracture treatment by ORIF aims at (1) primary stability of the
fracture in order to initiate fracture healing under some sort of
functional movement, (2) secondary stability in order to enable bony
consolidation, (3) correct alignment and adequate fracture reduction
in order to avoid malalignment and inadequate loading of joints, and
finally (4) a mechanical environment which promotes bone formation
and prevents delayed union or non-union.
Primary stability
Several biomechanical principles can be employed to achieve
sufficient primary stability in osteoporotic bone. As we have seen
earlier a critical point in fracture fixation of osteoporotic bone is the
interface between implant and bone. Thus, internal fixation devices
that allow load sharing with host bone should be chosen to minimize
stress at the bone
–
implant interface. This can be achieved by employ-
ing fixation devices which have a maximum of contact area between
implant and bone. Examples are long plates and nails with many
locking options or plates with a larger surface area providing more
possibilities for screw placement. Plates with a larger contact area
effectively reduce the local compressional strain on the bone. Similarly,
more thinner screws generate smaller local strain in cortical as well as
trabecular bone compared to fewer thicker screws [45]. Thinner screws
have the additional advantage of providing more flexibility and thus
the ability to distribute the load within a larger volume of bone. The
advantages of locked plates over conventional plates in providing
better stability have been discussed earlier in this manuscript.
Secondary stability
Secondary stability can only be achieved if sufficient primary
stability is provided. In addition bone fatigue by brittle failure, creep or
trabecular crushing has to be prevented. The limiting factor for
secondary stability is the limited fatigue strength of osteoporotic
bone. As bone fatigues at locations of high strain the primary principle
of secondary stability is the prevention of excessive strain and strain
concentrations. Thus, as mentioned before, implants which distribute
the strain over a larger area by large surfaces or by more screws or bolts
may prevent bone from early fatigue. Also loading which would
generate excessive strains locally must be avoided. Thus, implants with
additional features such as anti-rotation or anti gliding mechanisms
can potentially prevent excessive shear or tensile loads [46]. Examples
C. von Rüden, P. Augat / Injury, Int. J. Care Injured 47S2 (2016) S3
–
S10
S5