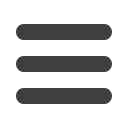
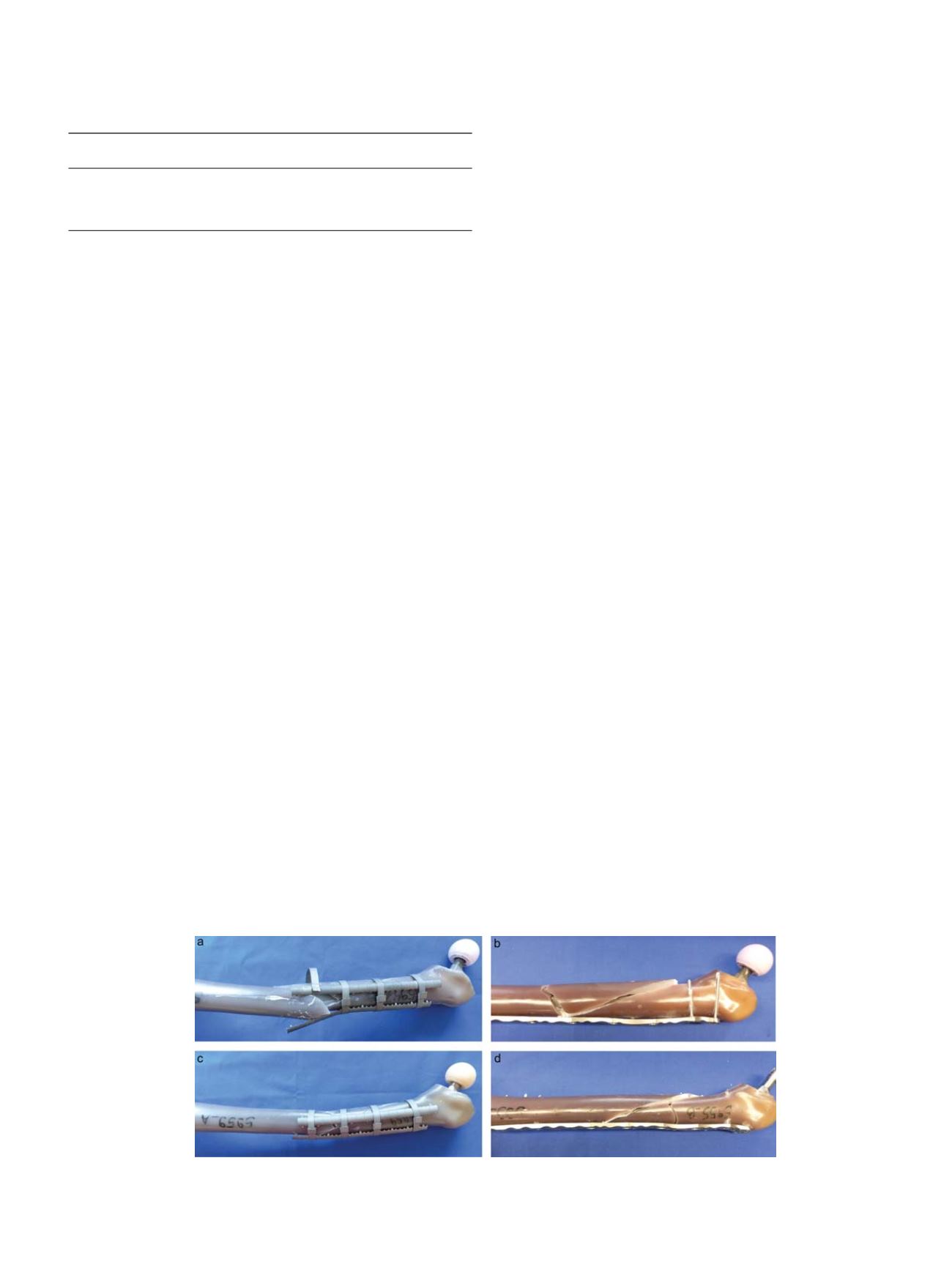
While the differences in the stability of the osteosynthesis were
apparent with a short stem implanted, the long stem increased
stiffness and strength such that differences between osteosynthesis
techniques were no longer discernible. The influence of stem length on
the performance of cemented hip-arthroplasty was also investigated
by Moroshima et al. 2013. Their results showed that sawbones break at
a statistically significantly lower torque to failure when a shorter stem
is used in comparison to a stem with a conventional length and the
same offset [31]. In 2011, Rupprecht et al. determined that the femoral
stem itself significantly reduces the fracture strength by 32%. But in
their study a cemented THA was investigated [30]. In 2014, Moazen
et al. used a finite element model to demonstrate that in treatment of
B1 fractures, a single locking plate can be used without complications if
partial weight bearing is followed. In the case of B2 fractures, long stem
revision and bypassing the fracture gap by two femoral diameters is
recommended. But considering the risk of single plate failure, long
stem revision could be considered in all comminuted B1 and B2
fractures. Double plating was also described as an alternative [7,34].
Clinical data suggest a higher failure rate for ORIF in B1 fractures
compared to the revision in the case of B2 fractures. In clinical practice
the intraoperative evaluation of the classificationwithin the Vancouver
system is very difficult and often leads to wrong results. If a B2 fracture
is treated like a B1 fracture, failure of the osteosynthesis can follow
frequently [35].
The present study has its strengths and limitations. To our
knowledge it was the first study simulating a clinically characteristic
fracture with an obtuse fracture angle. The aim of our study was to
investigate a clinically typical fracture pattern because the effective-
ness of fracture fixation is also dependent of the fracture location and
fracture angulation. Leonidou et al. developed a simplified parametric
finite element model of a cemented total hip replacement for the
management of Vancouver B1 fractures. Through the evaluation of
different fracture angles they found, that for poor bone quality and
obtuse fracture angles, alternative management methods such as
single locking plates might be required as the fixation might be under
higher risk of failure [36]. To our knowledge it was also the first
biomechanical study to compare the Gundolf cerclage system (CCG)
with a locking plate system for the management of Vancouver
B1 fractures. Compared to other cerclage systems the CCG system has
a broad contact surface which could be responsible for different
biomechanical performance. Another unique feature of the CCG system
is the presence of spikes along the stabilizers, which penetrate the
cortical bone and should prevent the CCG system from slipping out
of position. There was no breakage of the cerclages in the CCG
system during testing. In contrast, breakage of cable wires is reported
frequently.
As a limitation of this study it was not possible, based on the test
conditions of synthetic femurs, to investigate the cutting of cerclages
into the bone. It is observed that common cable wires can cut into the
bone. This problem has not been noticed with the use of the CCG
System in former clinical studies [24,37]. It was described that the CCG
System allows for controlled compression of the titanium bands. A
previous study of Lindtner et al. with histological investigations three
weeks postoperatively showed that the osteoblastic line on the inner
side of the titanium band and the surface of the femur provides
evidence of the tendency towards union or bone healing. There was no
evidence of necrosis, although the titaniumbandwas positioned firmly
on the bone, which was demonstrated in a microradiography [24,37].
The broad contact face of the titanium bands is intended to not
constrict the bone and to not disrupt the blood flow. The intention of
the stabilizers is to give initial stability to the bone and strengthen
it through osseointegration of the rough titanium surfaces [38].
As mentioned above, it was not possible to investigate biological
conditions within this biomechanical study.
We decided to use synthetic femurs because they have less
interspecies variability of physical properties than bone of human
donors. This increases comparability and avoids inherent variability in
bone quality, geometry and the potential presence of preexisting
damage [35,39, 40]. Recent industrial developments and an increasing
number of mechanical tests have led to the development of synthetic
bones with similar mechanical qualities to human bones. The
sawbones fourth generation synthetic femurs used in our study have
been used in many previous studies. The mechanical properties of
these femurs are well known and these synthetic bones are the most
similar to real bones that are used in in vitro mechanical tests [41].
However, as periprosthetic fractures typically occur in bones of elderly
individuals with diminished material, properties, and increased
fragility, the sawbone specimens may result in an overestimation of
the load to failure and consequently in the number of load cycles to
failure. Sawbones might also have better screw purchase than normal
bone. Although the sawbone specimens were considerably stronger
compared to human bone we could not produce any plate breakage, in
contrast to previous biomechanical studies [42]. Also no pullout of the
screws has been observed neither with the proximal screws (4 mm
cortical) nor with the distal screws (5 mm cortical).
Table 1.
Relative movements of the stem and fragments in axial direction after 1000 cycles
(mean ± SD).
Movement proximal to
distal fragment [mm]
Movement stem to
proximal fragment [mm]
Long stem/cerclage
0.1 ± 0.1
1.1 ± 1.1
Long stem/plate
0.2 ± 0.1
0.3 ± 0.2
Short stem/cerclage
0.8 ± 0.1
3.2 ± 1.1
Short stem/plate
4.1 ± 1.8
0.5 ± 1.7
Fig. 6.
(a) Typical Breakage of the
“
long stem/cerclage
”
samples. (b) Typical Breakage of the
“
long stem/plate
”
samples. (c) Typical Breakage of the
“
short stem/cerclage
”
samples. (d) Typical Breakage of the
“
short stem/plate
”
samples.
K. Gordon et al. / Injury, Int. J. Care Injured 47S2 (2016) S51
–
S57
S55