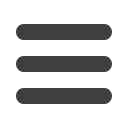
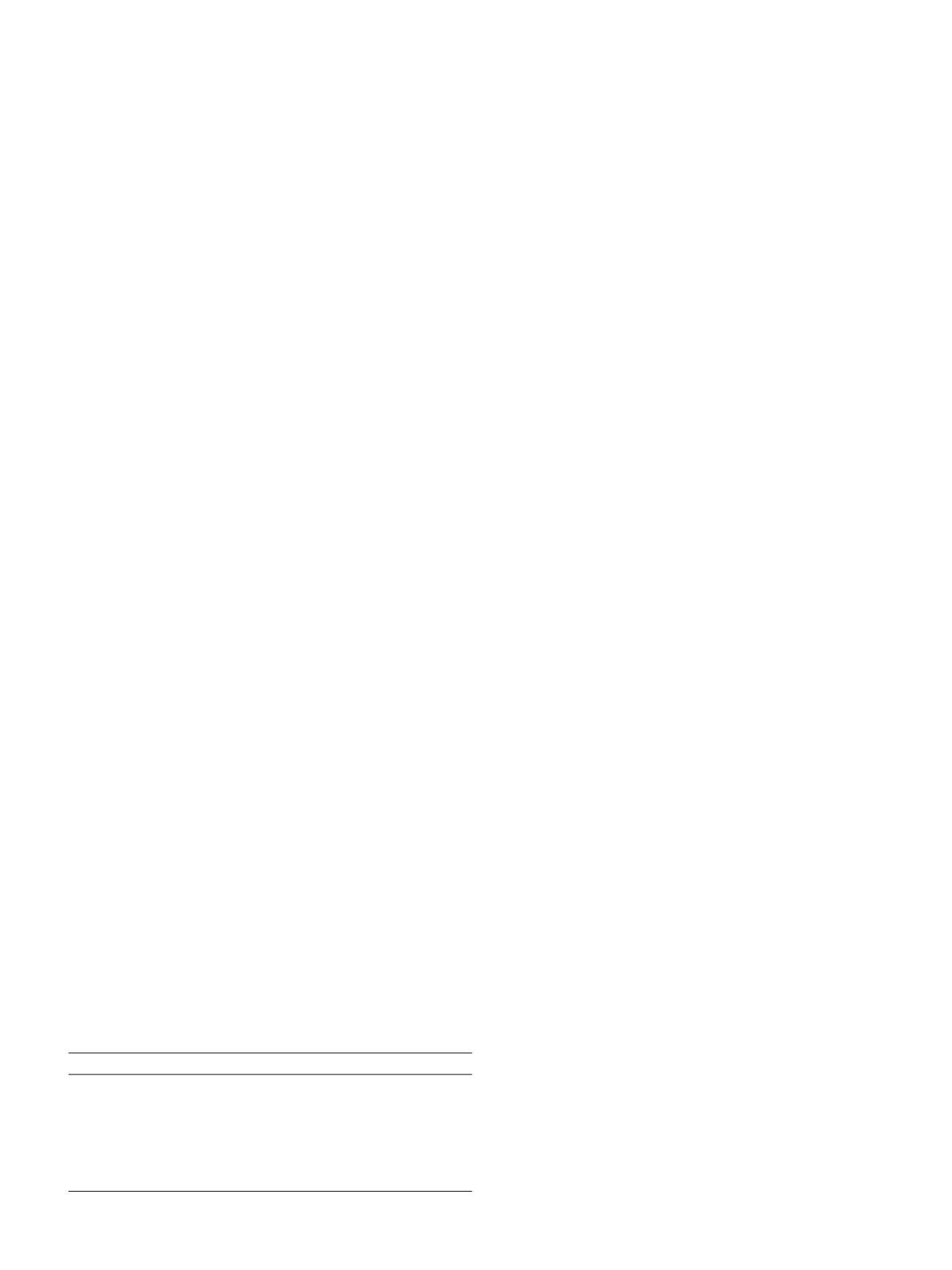
Introduction
Osteoporosis and particularly osteoporotic fractures have a high
impact both on the quality of life of patients and on the financial
aspects of Western health care systems. Biomaterials have gained
interest to enhance bone healing in osteoporotic fractures and to
improve treatment outcome [1].
Among many other materials hydroxyapatite and namely nano-
particulate hydroxyapatite is a potential candidate as bone substitute
material in osteoporotic bone for improvement of bone healing due to
its osteoconductive effects. Nanoparticulate hydroxyapatatite with
needle shaped HA crystals with a size of around 20 nm has already
been investigated in experimental and clinical settings for dental and
orthopaedic applications [2
–
10]. In general, good new bone formation
via osteoconductivity with this type of nanoparticulate hydroxyapatite
was reported. In all the above mentioned studies physiological and
not osteoporotic bone was investigated.
Collagens represent 25
–
35% of the total body proteins and can be
found in cartilage, bone and in almost all types of soft tissue [11].
Among 28 known different collagen types, collagen type-I is the most
abundant in the body and in bone representing more than 90% of the
organic mass in bone [12]. Collagens contain sections with the amino
sequence arginine, glycine, aspartic (RGD) which was discovered as a
small peptide ligand with high affinity to integrins increasing the
adhesiveness of surface implants for osteoblasts via binding to those
transmembrane integrin receptors [13]. Facilitated cellular attachment
of pre-osteoblasts on collagen via RGD-peptides with theoretical
enhancement of new bone formation is therefore of interest in the use
of collagens in composite biomaterials [14].
The intention of the current study is to assess new bone formation
and degradation behavior of nanocrystalline hydroxyapatite with or
without collagen-type I in osteoporotic bone defects in metaphyseal
bone defects in osteoporotic goats. The hypothesis is that both
nanoparticulate hydroxyapatite and nanoparticulate hydroxyapatite
with collagen-type I enhance new bone formation compared to
empty control defects and that the additional use of collagen type-I
improves new bone formation compared to plain nanoparticulate
hydroxyapatite.
Materials and methods
Study design
There were three different treatment groups: group I: empty
defect, group II: nanoparticulate hydroxyapatite, group III: nanoparti-
culate hydroxyapatite + collagen type I. In three osteoporotic Chinese
mountain goats, a total of 24 bone defects were created that were
either filled with nanoparticulate hydroxyapatite or nanoparticulate
hydroxyapatite + collagen type I or were left empty after randomisation
(Table 1). In each animal, 2 defects in the left iliac crest, 2 defects in the
right iliac crest, 1 defect in the left distal femur, 1 defect in the third
lumbar vertebra, 1 defect in the fourth lumbar vertebra and 1 defect in
the fifth lumbar vertebra were created with a total of 8 defects per
animal. Animal Research Ethics approval was obtained from the
Animal Experimentation Ethics Committee of the Chinese University of
Hong Kong before start of surgeries (Ref: 08/029/MIS).
Nanoparticulate hydroxyapatite
The hydroxyapatite (HA) used in the present study is a fully
synthetic injectable nanocrystalline paste (Ostim
®
, aap Biomaterials
GmbH, Dieburg, Germany) and consists of a suspension of pure
hydroxyapatite in water prepared by a wet chemical reaction. The
needle shaped HA crystals with a size of 21 nm in a-direction and of
36 nm in c-direction form agglomerates. Phase purity of the HA was
determined by X-ray-diffraction which shows conformity with pure
HA and an average crystallite size of 18 nm. The atomic ratio of calcium:
phosphorus is 1.67. Ostim
®
paste does not harden after application into
the bone and is free of endothermical heating in contrast to calcium
phosphate bone cements.
Collagenwas derived from split skin of pigs and purified by a multi-
stage process including acidic and alkaline treatment. Precipitated
hydroxyapatite was prepared in a suspension of purified collagen to
which phosphoric acid and calcium oxide were added under constant
stirring. The composite was dried, milled and mixed with pure
hydroxyapatite to yield a composite containing hydroxyapatite and
collagen a ratio of 80/20 and a solids content of 34.5
All materials were filled in 2 ml syringes and sterilized by gamma-
irradiation.
Animals
According to previously established animal model [15
–
17], three
female skeletally mature Chinese mountain goats were used for this
study. The ages of the animal were at least 3 years old and skeletal
maturity was confirmed by growth plate closure at the distal femora
and proximal tibia by radiography. The goats were housed in air-
conditioned and dark-light cycle-controlled partitions and were cared
for by qualified veterinarians at the Laboratory Animal Service Centre,
The Chinese University of Hong Kong, during the entire study.
Anaesthesia
All surgical operations were performed under general anaesthesia.
Sedation was introduced by a mask at 5% isoflurane (VCA ISO,
Halocarbon Laboratories, South Carolina, USA), immediately followed
by standard tracheal intubation using a laryngoscope. Maintenance
was kept at 1
–
2% of isoflurane with respiration monitored (apAlert
RM5, MBM, Coorparoo, QLD, Australia) throughout all procedures
including ovariectomy operations, Bone Mineral Density (BMD)
scanning and bone defect creation [15,17,18].
Induction of osteoporosis by ovariectomy and low-calcium diet
Bilateral ovariectomy was performed under general anaesthesia
with standard aseptic surgical technique. Postoperatively, all animals
received regular analgesics with a 0.5 ml intramuscular injection of
Temgesic (Reckitt & Colman Products, Ltd., Hull, UK) every 6 h for 2
days. The ovariectomized goats were fed with a low-calcium diet
containing 50% of food pellet with 0.2% calcium (Glen Forrest
Stockfeeders, Glen Forrest, Australia) plus 50% Wheaten Chaff with
0.3% calcium (O
’
Driscoll, Greerock, Australia) after the operation.
All goats were kept for 6 additional months until development of
osteoporosis prior to receiving bone defect creation. Osteoporosis was
confirmed by BMD scanning by peripheral quantitative computed
tomography (pQCT, Stratec, XCT2000L, Germany) at each calcaneus
according to our established protocol [15].
Table 1
Study design with treatment protocol for each of the 24 defects.
Goat 1
Goat 2
Goat 3
Right iliac crest #1
Empty
HA
HA + Col-type-I
Right iliac crest #2
Empty
HA
HA + Col-type-I
Left iliac crest #1
HA
HA + Col-type-I
Empty
Left iliac crest #2
HA
HA + Col-type-I
Empty
Left distal femur
Empty
HA
HA + Col-type-I
Lumbar vertebra 3
HA
HA + Col-type-I
Empty
Lumbar vertebra 4
HA + Col-type-I
Empty
HA
Lumbar vertebra 5
Empty
HA
HA + Col-type-I
V. Alt et al. / Injury, Int. J. Care Injured 47S2 (2016) S58
–
S65
S59