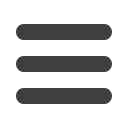
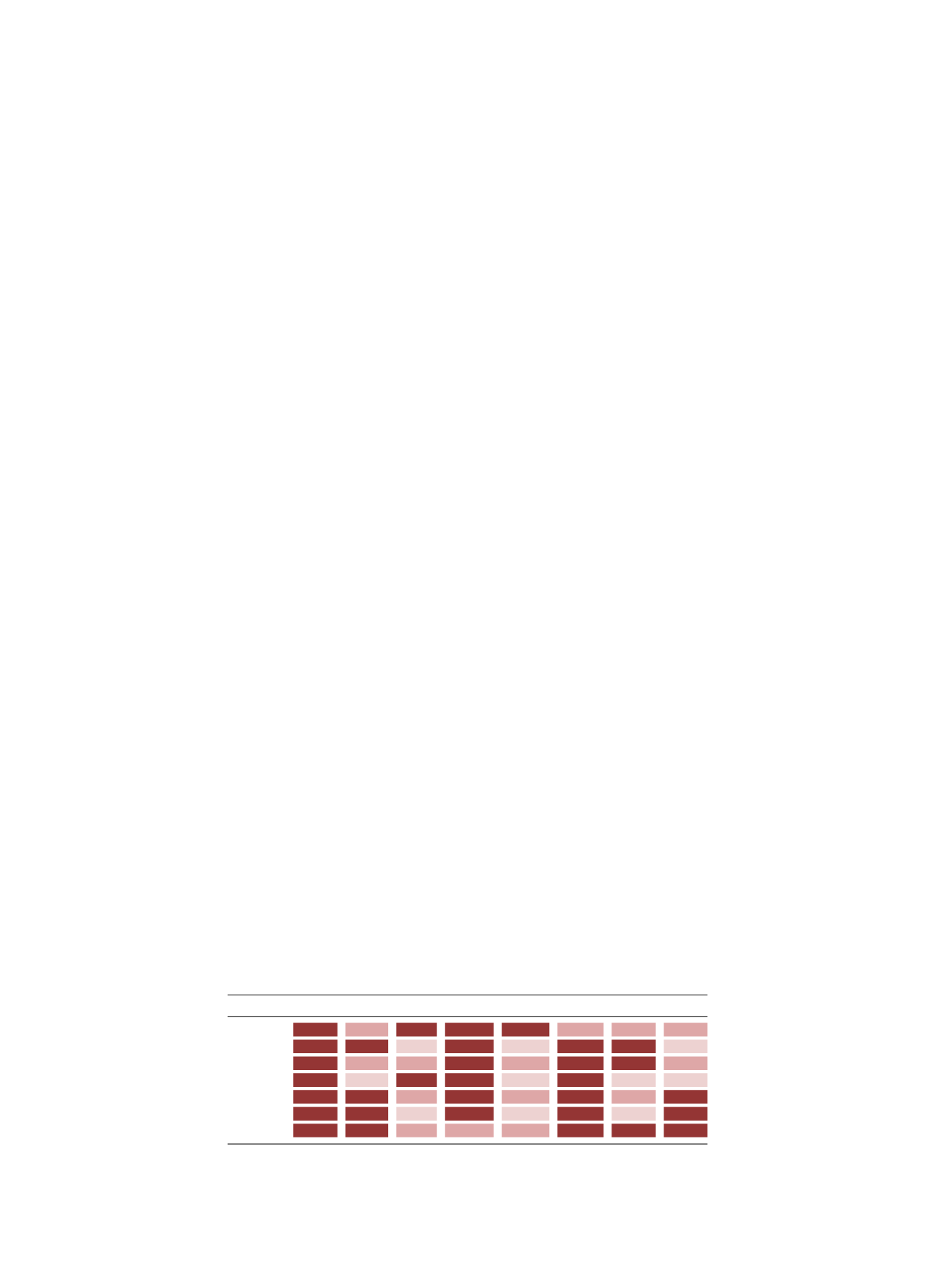
which is critical for avoiding subsidence. Material hardness is another
relevant property that impacts shock absorbance in the subchondral
bone. If a material is too hard, the mechanical environment for the
overlying cartilage might be negatively affected. Even though the
compressive properties are important, a fracture site is also subject to
shear and bending forces. When using materials with low bending and
shear resistance, it is necessary to use screws or other hardware to
neutralize these forces to provide a mechanical construct that can
withstand not only compression forces, but also shear and bending
forces. When using bone graft substitutes, the available materials
possess a wide variety of biological properties, which range from non-
resorptive to gradually remodeling, to rapidly degrading characteristics.
Bone graft substitutes should cause no or a very limited inflammatory
reaction in the surrounding tissues following implantation.Whilemany
materials are biologically inert, it is common for many classes of
materials to exhibit some osteoconductive propertieswhile fewprovide
an osteoinductive effect. The preferred material for a given clinical
situation depends on a number of factors, which include type of injury,
patient age, physical demands, bone quality, and surgeon experience.
Composites for augmentation
A variety of biomaterials are used for a range of clinical purposes,
including augmenting vertebral bodies to resist axial loading, reinfor-
cing implants at the bone-implant interface, and filling voids created by
osteotomies, resections, and reconstructions. The characteristics of
biomaterials are increasingly being individualized and are also starting
to incorporate patient-specific parameters. Properties for an ideal bone
substitute include: (1) void filling capacity; (2) structural support; (3)
osteoconductivity; (4) osteoinductivity; (5) osteogenicity; (6) minimal
morbidity; (7) cost-effectiveness; and (8) unlimited availability. There
is currently no bone substitute that fulfills all of these requirements,
and substitutes should be chosen based on the most critical need
when treating a particular fracture (Table 1). In general it seems
reasonable that the material should be replaced by bone over time.
However, when used in osteoporotic fractures, mechanical competence
over time is far more important than remodeling. This means that for
osteoporotic fractures the most important characteristics for the ideal
substitute seems to be offering mechanical stability while remodeling
and replacement by host bone seems less important. As a result, the
research field of augmentation composites is expanding rapidly. Of the
various bone graft substitutes available at present, injectable calcium-
phosphate compounds are by far the most widely documented for use
in tibial plateau fractures. Based on published studies it seems that
calcium-phosphate cement can be a good alternative to bone grafting
for filling of a subchondral void in tibial plateau fractures. This section
will summarize some of the recent developments in new composite
technology and provide an overview of the available composites for
osteoporotic bone augmentation.
Hydroxyapatite
In fractures that involve a metaphyseal defect, such as is present in
many tibial plateau fractures, preformed blocks of hydroxyapatite can
be used to fill the void. In a study by Bucholz et al. [34] published
in 1989, forty patients with tibial fracture fractures were randomized
to filling of the subchondral void with either contoured porous
hydroxyapatite blocks combined with hydroxyapatite granules or
autologous cancellous bone harvested from the ipsilateral iliac
crest. All patients underwent conventional screw and plate fixation.
Radiological and clinical assessments up to an average of 35 months
did not reveal any significant differences between the two groups.
Biopsies at the time of planned hardware removal in 7/20 patients in
the hydroxyapatite group showed incorporation of the hydroxyapatite
block by bone ingrowth with close apposition of new bone against the
implanted block. Therewas no apparent evidence of implant resorption
or inflammatory activity. The authors concluded that porous hydroxy-
apatitewas an excellent osteoconductive scaffolding for bone ingrowth,
and that the biodegradation occurred at an extremely slow pace.
PMMA
Injectable materials that harden in-situ, such as standard poly-
methylmetacrylate (PMMA), can be used to improve screw purchase in
osteoporotic bone, fill subchondral voids in tibial plateau fractures, and
augment metaphyseal fracture stabilization at other anatomical loca-
tions. Even though PMMA has been used successfully for augmentation
in osteoporotic fractures, including hip and wrist fractures, [35
–
37],
some clinicians still have concerns about using PMMA foraugmentation
in the treatment of fractures of the extremities. The perceived potential
drawbacks include anexothermic reactionduring curing, inabilityof the
cement to be remodeled, risk of inhibiting fracture healing if interposed
between fracture surfaces, and difficulty in removing the cement if
revision surgery becomes necessary.
Temperature considerations in PMMA augmentation
PMMA polymerization may lead to the development of supraphy-
siological temperatures and harm the surrounding bone tissue and
cartilage. In one basic research study, temperature was monitored in
the proximity of in-situ PMMA augmented screws, the subchondral
bone, and on the articular surface during augmentation of four screws
in the humeral head [38]. Overall, only small temperature increases
were reported. The temperature increase was highest at the screw tips
and decreased with increased distance from the cement. The
maximum temperature measured on the articular surface during
polymerisation was 38.3°C. The highest temperature at the subchon-
dral bone was 43.5°C, which is well below the stated threshold for
necrosis and apoptosis of bone tissue in the literature.
Table 1.
Characteristics of bone augmentation materials.
Void filler Structural Inductive Conductive Osteogenic Low morb.
Low cost Unlimited
ATBG
S-ALG
NS-ALG
DBM
CaP
CaS
PMMA
ATBG = autologus bone graft, S-ALG = Structural Allograft, NS-ALG = Non Structural Allograft, DBM =
Demineralized Bone Matrix, CaP = Calcium Phosphate, CaS = Calcium Sulfate, PMMA = Polymethylmethacrylate.
Dark Pink = Strongly Advantageous; Salmon = Weakly Advantageous; Light Pink = Not Advantageous.
C. Kammerlander et al. / Injury, Int. J. Care Injured 47S2 (2016) S36
–
S43
S40