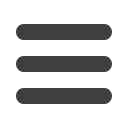
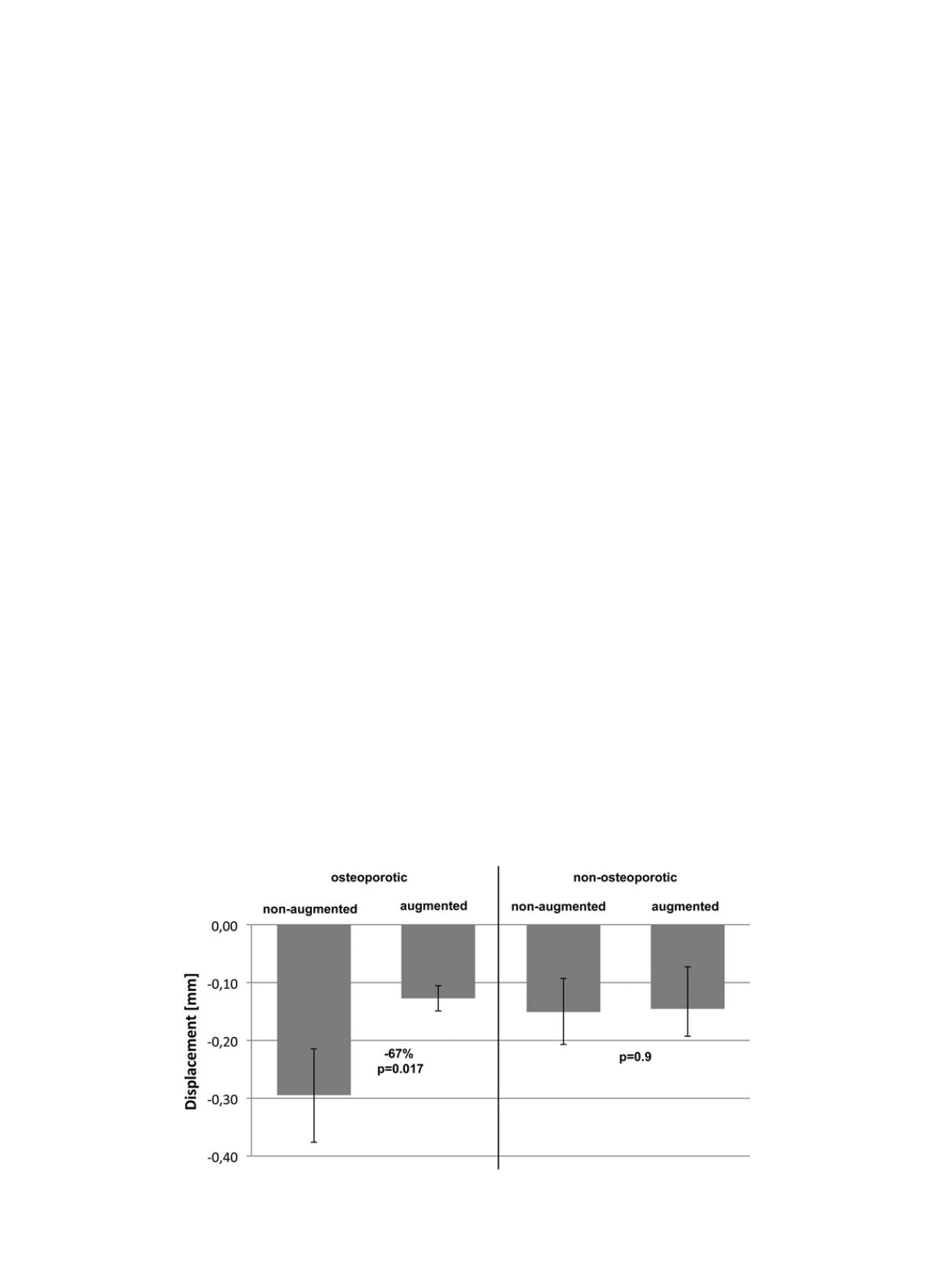
Implant augmentation has been shown to increase anchorage between
the implant and the bone, additionally it can rule out the influence of
osteoporosis. Certain biomechanical studies could proof the benefit of
augmentations techniques in osteoporotic fracture fixation [60
–
62].
Clinically implant augmentation was introduced for the treatment
of osteoporotic proximal femur fractures and proximal humerus
fractures [63].
In our first study we investigated the potential of implant
augmentation in the treatment of osteoporotic distal femur fractures
[11]. Therefore we used 12 custom made artificial osteoporotic bone
models of the distal femur. In both groups (
N
= 6 per group) an AO 33
A3 extraarticular fracture with a fracture gap of 15 mm (representing a
comminution zone) was created. The proximal part of the femur was
replaced by a 3
rd
generation composite bone (Sawbones, Malmö,
Sweden). Fixation in the proximal part was performed in a rigidmanner
to focus biomechanics on the distal/metaphyseal fixation. Fracture
fixation was performed using a standard angular stable plate (locking
compression plate for the distal femur, LCP DF, DePuy Synthes,
Solothurn, Switzerland). For distal fixation seven 5 mm self-tapping
locking screws were used. In the augmented group prior to screw
insertion 1 ml of PMMA based (polymethylmetacrylate) bone
cement (Traumacem V+, DePuy Synthes, Solothurn, Switzerland)
was injected using a side opening cannula. Cement injection was
performed into the medial part of the bone samples from dorso-caudal
to anterior-caudal. After cement curing biomechanical testing was
performed using a servohydraulic material testing machine (Instron
8874, Instron, High Wycombe, Bucks, United Kingdom). Cyclic axial
loading was performed with a frequency of 2 Hz and a peak load of
500 N for 45,000 cycles. Afterwards specimens were loadedwith 750 N
peak load until failure.
From the test machines transducers system time, cycle, axial
load and axial displacement were recorded with a frequency of 50 Hz.
Axial stiffness was calculated from the load displacement curves.
Furthermore, the displacement was calculated for selected cycles.
The mean axial stiffness was 102.5 N/mm in the non-augmented
group compared to 139.7 N/mm for the augmented group. This
difference was statistically significant with
p
= 0.04. The displacement
after 45,000 cycles was significant lower for the augmented group
(0.68 mm) compared to the non-augmented (2.28 mm;
p
= 0.001).
The results of this study showed a promising potential of locked
plate augmentation as an option in the treatment of severe osteopor-
otic distal femur fractures. The augmented group showed significantly
higher axial stiffness and less displacement as well as a significant
higher number of cycles until failure. From these results we concluded
that implant augmentation has the potential to increase construct
stability and therefore can reduce complication rate (secondary loss of
reduction, implant loosening).
In the second study we investigated the influence of bone quality
on the effect of implant augmentation [64]. In several biomechanical
studies using human osteoporotic specimens augmentation has been
shown that the lower the bone mineral density, the greater the
advantage of augmentation [60,61]. In the previously performed
investigation we found a significant reduction of cut-out due to
augmentation in artificial osteoporotic femora. The aim of this second
study was to investigate the influence of bone quality on the impact of
augmentation in a distal femoral fracture model. Therefore, we used
8 artificial osteoporotic and 8 non-osteoporotic specimens of the distal
femur; 4 of each quality were augmented and 4 not. The implants,
instrumentation, test-setup and biomechanical testing was equal to
the first study. Additionally a 3D motion tracking system (Optotrak
Certus Motion Capture System; Northern Digital Inc., Waterloo,
Canada) was used to determine interfragmentary movements.
The mean axial stiffness was comparable within both, the non-
augmented (osteoporotic 103 N/mm (SD 17) vs. non-osteoporotic
103 N/mm (SD 12;
p
= 0.944)) and augmented groups (osteoporotic
140 N/mm (SD 23) vs. non-osteoporotic 136 N/mm (SD 28;
p
= 0.845)).
Augmentation therefore increases axial stiffness significantly about
36% in the osteoporotic group (
p
= 0.043) and not significantly about
32% in the non-osteoporotic group (
p
= 0.084). The mean displacement
was significant lower for the augmented osteoporotic group after
45,000 cycles compared to the non-augmented osteoporotic group
(
p
≤
0.017; Figure 5). Augmentation reduced cut-out in the osteopor-
otic specimen about 67%. In the non-osteoporotic group displacement
showed no statistical significant difference, the augmentation showed
no influence to the cut-out of the screws after 45,000 cycles (
p
≥
0.9).
Furthermore, the screw removal torque was measured after biomech-
anical testing. With 3.3 Nm (SD 0.84) the augmented group showed a
significant higher screw removal torque. In the non-augmented group
a mean torque of 1.9 Nm (SD 0.93) was necessary to remove the screws.
These 72% increase in peak torque were found to be statistically
significant (
p
≤
0.01) but no problems occurred during screw removal.
The maximum torque measured was 6.1 Nm. In this study implant
augmentation significantly increases mechanical stability in osteopor-
otic bone. Mechanical stability was comparable to non-osteoporotic
bone model; therefore, implant augmentation has the potential to rule
out the influence of osteoporosis in the treatment of distal femoral
fractures. We found no biomechanical benefit of augmentation in non-
osteoporotic bone samples.
Fig. 5.
Mean cut-out in mm after 45,000 cycles for the osteoporotic and non-osteoporotic specimens with standard deviation.
M. Lenz et al. / Injury, Int. J. Care Injured 47S2 (2016) S44
–
S50
S48