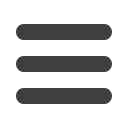
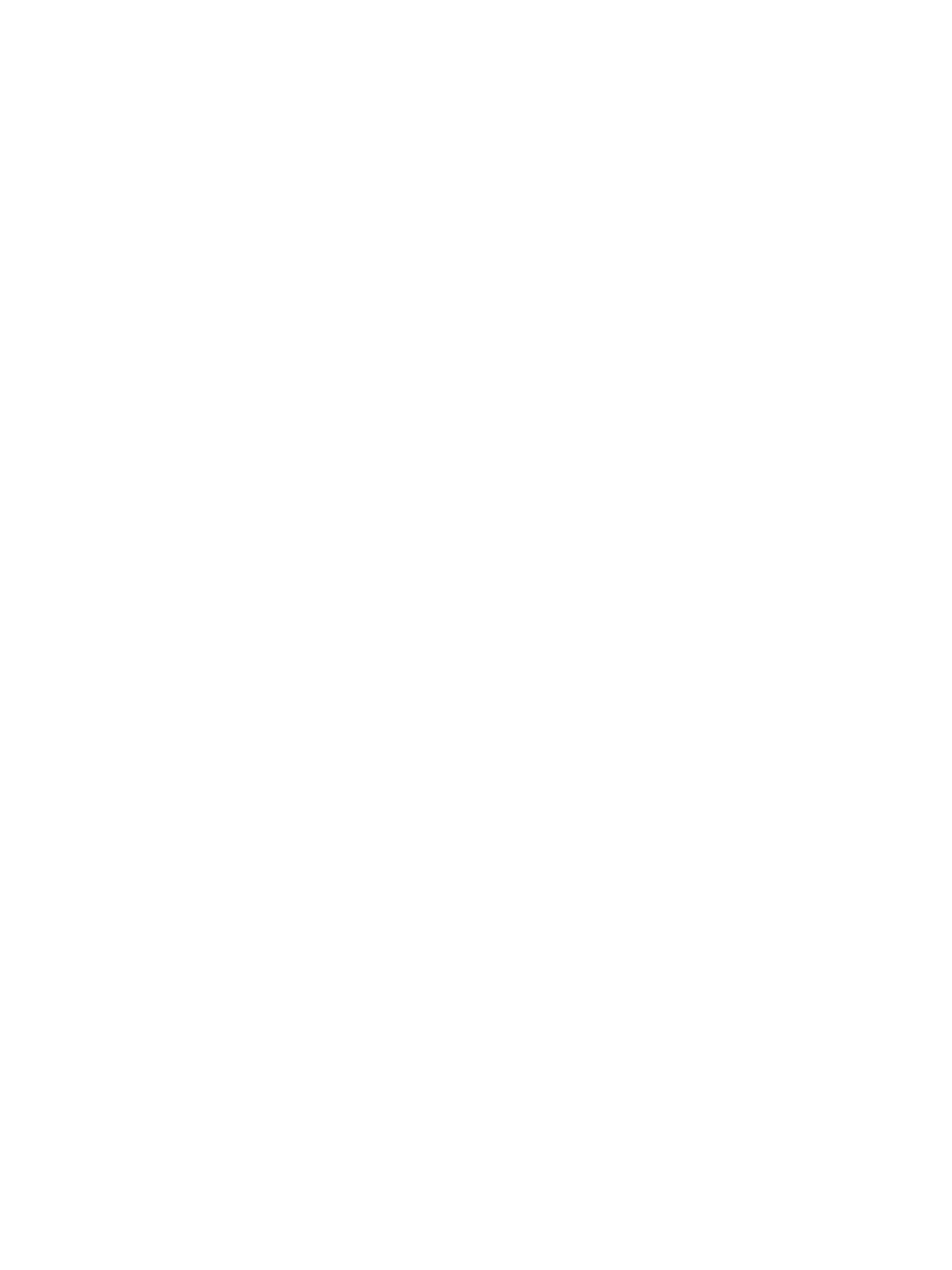
which compose 10% of bone
’
s organic matrix, also may affect bone
mechanical properties. Osteocalcin stimulates mineral maturation,
inhibits bone formation, recruits osteoclast precursors to bone
resorption sites, and helps with their differentiation into mature
osteoclasts [107]. Osteopontin plays a role in mineralization and assists
the bone resorption process by anchoring osteoclasts to the mineral
matrix of the bone surface [88]. More importantly, these proteins have
been recently considered to act as the glue that holds mineralized
collagen fibers together. When a force is applied, these components
stretch, help dissipate energy by breaking sacrificial bonds between
adjacent collagen fibrils, and prevent harmful crack formation and
propagation [108]. Thus, alterations to the matrix composition of both
collagenous
and
non-collagenous proteins may alter bone biomech-
anical properties. Increased serum osteocalcin and osteopontin has
been reported in postmenopausal womenwith osteoporosis compared
to healthy controls [109,110].
In summary, there is increasing evidence of the role of bone
’
s
organic matrix on age- and disease-related changes in bone
’
s
mechanical properties. Enzymatic crosslinking of collagen is generally
considered to have a positive effect on bone
’
s mechanical properties,
while non-enzymatic crosslinking can lead to deteriorated bone
mechanical properties with aging and disease. Non-collagenous
proteins play a role in the prevention of harmful microdamage
formation. Though osteoporosis is generally defined as a loss of bone
mass, there are considerable matrix changes, particularly in collagen
crosslinks, which cause a loss of bone quality.
Conclusions
The bone
’
s inorganic and organic composition, its trabecular and
cortical nano-, micro-, and macroscopic architecture, and the hetero-
geneity of these structural features all have impact on age- and disease-
related changes in bone
’
s mechanical properties. Though osteoporosis
is generally defined as a loss of bone mass, there are considerable
changes of the structure and matrix itself, which can cause a loss of
bone quality.
It is known, that cortical bone plays a major role in determining the
mechanical competence of bone and the risk of fracture; the age-
related alterations of its geometrical features and its local porosity,
though, have long been poorly understood and underestimated. The
number of trabeculae in trabecular bone, trabecular thickness and the
degree of connectivity all influence the mechanical strength of a bone.
In osteoporosis a decrease of all these characteristics is seen. Especially
in bones with increased risk for osteoporotic fractures, however, the
remaining trabecular tissue is largely heterogeneous, with regions of
different mineralization, stiffness and strength.
Both, the trabecular and the cortical component undergo different
changes at different times. Bone remodelling occurs on osseous
surfaces and, thus, osteoporotic bone loss is a function of surface
available for bone remodelling. The bone loss in early osteoporosis is
mainly trabecular and with increasing age the bone loss becomes
primarily endo- and intracortical.
The knowledge about this evolution in matrix and structure in
osteoporotic bone and about the differences between trabecular and
cortical bone could help with predicting, avoiding and treating
osteoporotic fractures. Future clinical imaging techniques will have to
consider structural measures of cortical and trabecular bone rather
than focusing on bone mineral density alone. In prophylactic treatment
regimens, the aimed for therapeutic region (i.e. trabecular versus
cortical) and mechanisms of action within the cascade of bone
remodelling might have to be chosen according to the patient
’
s age
and the individual advancement of bone changes. Eventually, when a
fracture has occurred, the non-operative or surgical treatment has to
be guided by both: the personality of a patient and the personality of
their bone.
Conflict of interest
The authors report no conflict of interest related to the content of
the manuscript.
References
[1]
Cooper C, Atkinson EJ, O
’
Fallon WM, Melton LJ, 3rd. Incidence of clinically diagnosed
vertebral fractures: a population-based study in Rochester, Minnesota, 1985-1989. J
Bone Miner Res 1992;7:221
–
7.
[2]
Schwartz AV, Kelsey JL, Maggi S, Tuttleman M, Ho SC, Jonsson PV, et al. International
variation in the incidence of hip fractures: cross-national project on osteoporosis for
the World Health Organization Program for Research on Aging. Osteoporos Int
1999;9:242
–
53.
[3]
Tosounidis TH, Castillo R, Kanakaris NK, Giannoudis PV. Common complications in hip
fracture surgery: Tips/tricks and solutions to avoid them. Injury 2015;46(Suppl 5):S3
–
11.
[4]
Makridis KG, Karachalios T, Kontogeorgakos VA, Badras LS, Malizos KN. The effect of
osteoporotic treatment on the functional outcome, re-fracture rate, quality of life and
mortality in patients with hip fractures: a prospective functional and clinical outcome
study on 520 patients. Injury 2015;46:378
–
83.
[5]
Guerado E, Cruz E, Cano JR, Crespo PV, Alaminos M, Del Carmen Sánchez-Quevedo M,
Campos A. Bone mineral density aspects in the femoral neck of hip fracture patients.
Injury 2016;47(Suppl 1):S21
–
4.
[6]
Greenspan SL, Perera S, Nace D, Zukowski KS, Ferchak MA, Lee CJ, et al. FRAX or fiction:
determining optimal screening strategies for treatment of osteoporosis in residents in
long-term care facilities. J Am Geriatr Soc 2012;60:684
–
90.
[7]
Smith MG, Dunkow P, Lang DM. Treatment of osteoporosis: missed opportunities in the
hospital fracture clinic. Ann R Coll Surg of Engl 2004;86:344
–
6.
[8]
Kleerekoper M, Nelson DA. Which bone density measurement? J Bone Miner Res
1997;12:712
–
4.
[9]
Ammann P, Rizzoli R. Bone strength and its determinants. Osteoporos Int 2003;14(Suppl
3):S13
–
8.
[10]
Nordin M, Frankel VH. Biomechnics of bone. In: Nordin M, Frankel VH, editors. Basic
Biomechanics of the musculoskeletal system. 4th ed. North American: LWW;
2012. p. 472.
[11]
Seeman E, Delmas PD. Bone quality
—
the material and structural basis of bone strength
and fragility. N Engl J Med 2006;354:2250
–
61.
[12]
Burstein AH, Reilly DT, Martens M. Aging of bone tissue: mechanical properties. J Bone
Joint Surg Am 1976;58:82
–
6.
[13]
Carter DR, Hayes WC. Compact bone fatigue damage: a microscopic examination. Clin
Orthop Relat Res 1977:265
–
74.
[14]
Keaveny TM, Hayes WC. A 20-year perspective on the mechanical properties of
trabecular bone. J Biomech Eng 1993;115:534
–
42.
[15]
Galante J, Rostoker W, Ray RD. Physical properties of trabecular bone. Calcif Tissue Res
1970;5:236
–
46.
[16]
Dempster WT, Liddicoat RT. Compact bone as a non-isotropic material. Am J Anat
1952;91:331
–
62.
[17]
Mittra E, Rubin C, Gruber B, Qin YX. Evaluation of trabecular mechanical and
microstructural properties in human calcaneal bone of advanced age using mechanical
testing, microCT, and DXA. J Biomech 2008;41:368
–
75.
[18]
Carter DR, Schwab GH, Spengler DM. Tensile fracture of cancellous bone. Acta Orthop
Scand 1980;51:733
–
41.
[19]
Mizrahi J, Silva MJ, Keaveny TM, Edwards WT, Hayes WC. Finite-element stress analysis
of the normal and osteoporotic lumbar vertebral body. Spine (Phila Pa 1976)
1993;18:2088
–
96.
[20]
Holzer G, von Skrbensky G, Holzer LA, Pichl W. Hip fractures and the contribution
of cortical versus trabecular bone to femoral neck strength. J Bone Miner Res
2009;24:468
–
74.
[21]
Gluer CC, Cummings SR, Pressman A, Li J, Gluer K, Faulkner KG, et al. Prediction of hip
fractures from pelvic radiographs: the study of osteoporotic fractures. The Study of
Osteoporotic Fractures Research Group. J Bone Miner Res 1994;9:671
–
7.
[22]
Hepp P, Theopold J, Osterhoff G, Marquass B, Voigt C, Josten C. Bone quality measured by
the radiogrammetric parameter
“
cortical index
”
and reoperations after locking plate
osteosynthesis in patients sustaining proximal humerus fractures. Arch Orthop Trauma
Surg 2009;129:1251
–
9.
[23]
Zebaze RM, Ghasem-Zadeh A, Bohte A, Iuliano-Burns S, Mirams M, Price RI, et al.
Intracortical remodelling and porosity in the distal radius and post-mortem femurs of
women: a cross-sectional study. Lancet 2010;375:1729
–
36.
[24]
Svedbom A, Ivergard M, Hernlund E, Rizzoli R, Kanis JA. Epidemiology and economic
burden of osteoporosis in Switzerland. Arch Osteoporos 2014;9:187.
[25]
Edidin AA, Ong KL, Lau E, Kurtz SM. Mortality risk for operated and nonoperated
vertebral fracture patients in the medicare population. J Bone Miner Res
2011;26:1617
–
26.
[26]
Jacquot F, Letellier T, Atchabahian A, Doursounian L, Feron JM. Balloon reduction and
cement fixation in calcaneal articular fractures: a five-year experience. Int Orthop
2013;37:905
–
10.
[27]
Osterhoff G, Baumgartner D, Favre P, Wanner GA, Gerber H, Simmen HP, et al. Medial
support by fibula bone graft in angular stable plate fixation of proximal humeral
fractures: an in vitro study with synthetic bone. J Shoulder Elbow Surg 2011;20:740
–
6.
G. Osterhoff et al. / Injury, Int. J. Care Injured 47S2 (2016) S11
–
S20
S18